I’ve finally identified a thing I found in my mouth nearly 25 years ago. Unfortunately, solving that old puzzle has left me with a new one concerning a protozoan normally found in the hindgut of cockroaches and termites.
I ran across it in the fall of 1990. My wife was a first-year medical student and had borrowed a microscope to study samples of human tissue for a course in histology. In those days, I was more interested in poetry than protozoa, but I was even more interested in procrastination. So, one morning I put my doctoral thesis aside and took the loaner ‘scope for a spin.
Her prepared histology slides–fixed cells stained in lurid pinks and powder blues–did not hold my attention for long. I wanted to see something alive and wriggling. I’d read about dust mites, so I put some floor-fluff on a slide, but that was disappointing: nothing but hair, skin flakes and coloured cloth fibres. Then, I sampled some water from a soup pot that had sat in the sink overnight, which was a little better. There were bacteria there, thousands of them, jostling and jiggling in the milky broth. But bacteria are boring.
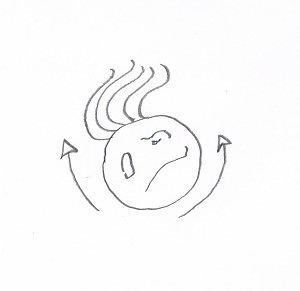
A reconstruction of The Thing. (The arrows are supposed to show how it moved. Yes, it looks like the cell has arms, but I don’t feel like drawing it again).
Finally, I swabbed some saliva from the back of my mouth and that was more like it. If you’ve ever done this, you’ll know what I found: big, ragged oral epithelial cells, swirling rafts of intriguing organic stuff, plump leukocytes that had recently eaten a bacterial meal. Then something truly bizarre floated into view. It was round and mottled, like a degenerate happy face with a crop of lank hairs sprouting from one side. It was rotating back and forth with a hypnotic, rocking motion: clockwise, then counterclockwise, then clockwise again. With each change of direction, the strands moved together in a languid arc.
Amazing! A parasite was living in my mouth, and it had hair like a rock star. What could it be? I grabbed a bus down to the McGill Life Sciences Library and pulled some parasitology texts from the stacks. From these, I determined that this creature had to be some kind of “flagellate” (the word was new to me). I leafed through the mug shots, blurred micrographs of Trichomonas, Chilomastix, Giardia, Retortamonas and all their troublesome colleagues, but I couldn’t find my guy anywhere. The known flagellate parasites were all were too pinched, or too pointy, and none had the lush head of hair I’d seen on my creature.
A couple of nights later, a burglar broke into our place and stole the microscope, which put an end to my amateur parasitology for a couple of decades. I went back to my real work, but never forgot that my mouth had miniature glam rockers living inside it. Years later, when I returned to microscopy (taking procrastination to a whole new level), the first thing I did was try to find those flagellates again. I swabbed my palate, scraped my tongue and spat on slide after slide, without success. By then, I had learned a lot more about protists, and I had acquired a good library of field guides and protozoology texts, none of which recorded anything quite like the organism I’d seen. I began to doubt my memory.
Then, just two days ago, a friend from Uruguay sent me a link to a video from Mexico, showing a presumed protozoan found in the bronchial lavage of a patient who had nearly drowned in a river. And there it was again, the Thing from my Mouth!
That rocking motion is exactly what I had seen in 1990. The video was posted to YouTube as an unknown “ciliado” (ciliate), and superficially it does resemble one. At first, it struck me as an overripe oligotrich, possibly bloated from osmotic pressure and moving poorly. But the way it was moving looked all wrong, even for an unhealthy specimen. That repetitive, ineffectual rocking movement was not like the familiar motion of the fused cilia (adoral polykinetids) that surround the cellular mouth of oligotrich ciliates, or any of the ciliary wreaths and fringes I knew from other free-living ciliophora. The rocking suggested something sessile that had come loose from its substrate, which reminded me that this “ciliate” had been found in bronchealveolar lavage fluid (BALF). As it happens, we all have ciliated cells lining some of our nasal and bronchial cavities, which perform the useful task of clearing mucus and debris from the lungs. The ciliated cells stay fixed in one place, and waste matter sort of “crowd surfs” over them, passing from one cell to the next on its way out the door. Could this be a detached bronchial cell?
A search within YouTube turned up another video, this one from the Diagnostic Bacteriology Laboratory at Singapore General Hospital. It shows a large clump of bronchial epithelium with the cilia busily working:
In this sample, the cells are all fixed in place and firmly attached to one another, so we don’t see the feeble rocking movement of the free-floating cell. Still, I’m persuaded me that the cells in both videos, as well as the one I’d seen back in 1990, are all ciliated nasal or bronchial cells.
And this brings me to the new puzzle. A comment on the second video sent me to an intriguing post at a pathology blog called Microcosm, where I learned something fascinating and, I’m sorry to say, a bit revolting. It seems there have been medical case reports of humans being infected by a lushly flagellated protozoan called Lophomonas blattarum, normally found living commensally in the intestines of cockroaches or termites.
L. blattarum has been known since 1860, when it was found and described by the great Samuel Friedrich Stein. Reports of respiratory infections in humans are more recent, dating back only as far as 1993 when the first case was reported in the Chinese Journal of Parasitology and Parasitic Diseases. Since then, many dozens of similar cases have been reported, which has lead to a growing belief, among some researchers, that Lophomonas blattarum constitutes “a potentially important cause of bronchopulmonary infection and respiratory symptoms.” (Martinez-Girón and van Woerden, 2013a).
The problem is, it might not be a genuine infection at all. In all of the published case reports of Lophomonas infection, the organisms were identified by light microscopy, either from living samples or stained preparations. To date, there have been no molecular studies of the purported parasite. Identifications have been based entirely on morphological features and symptomology.
One ultrastructural study has been done, but it does not support the theory that lophomoniasis is a genuine infective disease. The 12-author paper was published in Chinese, but from the English abstract (and by laboriously feeding the text to Google Translate one paragraph at a time), I gather that the researchers used optical and electron microscopy to examine mobile cells from BALF of 6 patients, which they found to be morphologically unlike Lophomonas. They also reviewed the existing literature on Chinese cases of lophomoniasis, and reached the following conclusion: “In the past 20 years, all the diagnosed cases as pulmonary Lophomonas blattarum infection reported in our country were misdiagnosed. Currently, there is no evidence to show Lophomonas blattarum as a pathogen resulting in pulmonary infection.” (Mu XL, et al., 2013) According to this study, the Lophomonas infections recorded in the literature were actually misidentified bronchial cells.
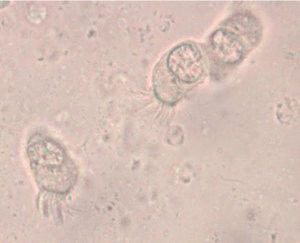
A typically murky micrograph of cells identified as Lophomonas blattarum in He et al, 2011. Martinez-Girón and van Woerden consider these to be misidentified bronchial epithelial cells.
Other workers, while agreeing that confusion between epithelial cells and Lophomonas has occurred, continue to maintain that many cases of infection are genuine. Martínez-Girón and van Woerden, who have published more about lophomoniasis than anyone, have responded to their Chinese colleagues by reaffirming their opinion that “the observation under light microscopy of this multiflagellated protozoon in symptomatic patients, who respond positively to antiprotozoal therapy, can reasonably be described as bronchopulmonary lophomoniasis.” (Martínez-Girón and van Woerden, 2013b)

The fine structure of Lophomonas blattarum (Beams et al, 1961, adapted from Kudo) . Note the wine-glass shaped calyx (CY), axial filament (AXF) and parabasal “collar” of tubules (PNT), all visible in the light microscope.
As someone who is usually more interested in the taxonomy of organisms than the pathologies they cause, I’m struck by the emphasis clinicians place on disease and symptoms rather than the creature itself. From a clinical point of view, the case for infection is strong: a given patient has respiratory symptoms, some apparently-flagellated cells are observed in bronchial lavage fluid, and the condition clears up with treatment by metronidazole, which is, after all, the preferred treatment for another metomonad parasite, Trichomonas vaginalis. These are all important facts that are hard to dismiss if you approach the subject from a clinical point of view. However, from a purely protistological perspective, we might note that identification by light microscopy is often unreliable, especially with such small cells; the micrographs in the published literature are usually quite poor, and may depict cells in an unnatural condition; the morphological characters used in these case studies tend to be weak, while stronger diagnostic characters, like the fine structures shown in the diagram to the right, are not mentioned; and, finally, the organism in question is a deep-branching anaerobe adapted to life in the intestines of insects of the order Blattodea, and it is a little suprising for it to flourish in a human lung. An organism’s habitat is a strong clue to its identity; this one is normally found in the airless hindgut of certain bugs, and possibly in the poop of certain bug-eating birds. Given that these flagellates, if that is what they are, were discovered in an untypical environment, a careful taxonomist might wait for more detailed morphological information, or, better yet, molecular data, before settling on a species-level identification. Where are the calyx and axial filament, which Richard Kudo managed to see in 1926? Where are the tubules of the parabasal body, which should encircle to the top of the cell like the ruffled collar of an Elizabethan gentleman? From what I’ve seen, they’re barely mentioned in the literature on lophomoniasis.
So we have a strong clinical profile combined with unimpressive taxonomic evidence, leaving us with a question that can’t be resolved until somebody succeeds in sequencing these cells. As Martinez-Girón and van Woerden conclude, “The development of a technique to culture the organism or the use of molecular techniques is required to resolve the issue.” If anybody is working on that, it will be interesting to see the results.
——————